The problem with looking at, say carbon , and accepting that this is its valence shell electron configuration:
is that it suggests not only that a single carbon atom will form only #2# covalent bonds normally, but also that its only means of forming a third bond would be to accept a lone pair of electrons from another atom in a coordinate bond. Now consider diamond, a tetrahedral macromolecular structure with #4# bonds per carbon atom:
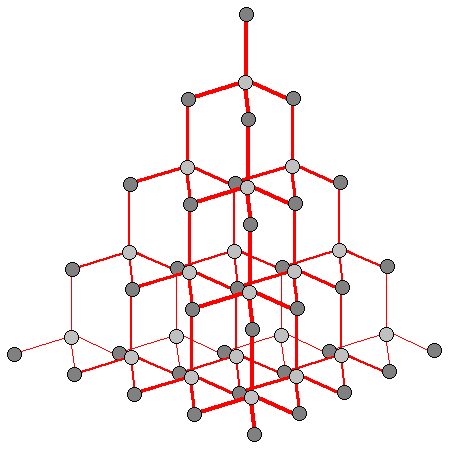
Well, you will notice that one of our valence orbitals is empty, whilst another is full; indeed, this discrepancy between the #2"s"# orbital and the #2"p"_z# orbital (as it tends to be denoted) is the very reason for our current predicament.
The solution is simple: during bonding, there is sufficient energy that a carbon atom will promote one of its #2"s"# electrons to occupy the #2"p"_z# orbital: this will leave four, half-filled atomic orbitals. Of course, this promotion requires energy beyond what an isolated carbon atom is capable of, even in a gaseous state, because this conflicts with the Aufbau principle. We say that the atom is excited:
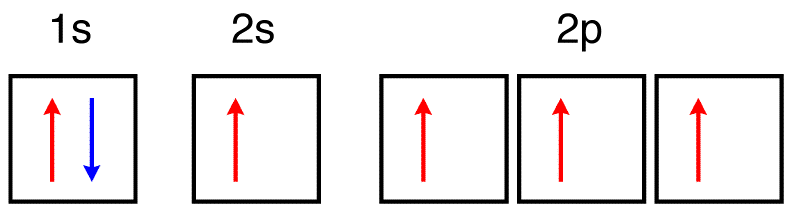
But this causes issues in itself. #"s"# orbitals and #"p"# orbitals overlap with orbitals on other atoms in different ways as a result of their shapes, and in fact the ability of #"p"# orbitals to overlap side-on is what is responsible for #pi# bonding - a necessary component of double and triple bonding. Yet diamond exhibits only single bonds.
We can also extend this issue further by associating it to bond length. If there were different possible combinations for orbital overlap throughout the structure, we would be looking at a structure with variable bond lengths, when we observe that all the #"C - C"# single bonds in diamond are #154"pm"# in length.

The solution to this problem is hybridisation. As stated in the answer section, this is the mixing of the atomic orbitals we've been dealing with up to now to form new hybrid orbitals that have two distinct characteristics:
- They are intermediate in nature between the types of atomic orbital used to form them.
- They are identical to one another.
In diamond #4# bonds are formed by a single carbon atom, and so we require #4# hybrid orbitals. Observe, then, that we have #4# atomic orbitals at our disposal. In hybridisation, the total number of atomic orbitals you put in, equates to the number of hybrid orbitals you get out; all that changes is the identity of the orbitals overall. Since we put in 1 #"s"# orbital alongside 3 #"p"# orbitals, so the outcome is #4# #"sp"^3# hybrid orbitals.
Don't forget that this all occurs during the process of bonding! Sticking with our diamond analogy, each carbon atom will now form #4# single covalent bonds with #4# other carbon atoms which, as shown in the diagram, gives us the familiar tetrahedral geometry that we observe diamond to have.
To conclude, then, this also allows us to explain why all of the #"C - C"# bonds are single bonds, and why they all have the same bond length: it is because the bonds that are formed are all due to the overlap of #"sp"^3# hybrid orbitals, and so they are all identical.